A New Hypothesis from an International Team of Researchers Led by CSFK's Senior Research Fellow May Explain the Formation of Earth-like Planets
An international team of scientists led by Zsolt Regály, Senior Research Fellow at the Konkoly Thege Miklós Astronomical Institute of the ELKH Research Centre for Astronomy and Earth Sciences (CSFK), has discovered a physical process that could lead to the creation of a multitude of planetary embryos. The new planet formation hypothesis may explain, among other things, how Earth-like planets or giant planets the size of Jupiter were formed. The discovery was reported in the prestigious Monthly Notices of the Royal Astronomical Society, the journal of the Royal Astronomical Society of England.
If you look up at the sky, you can see countless bright stars twinkling above your head. Perhaps you wouldn't even think that there are many more planets than stars. Most of these distant planetary systems are structurally very different from our own Solar System. Our current scientific knowledge suggests that existing hypotheses on the creation of planets are not entirely correct. How is it possible that, although planets are the most common objects in the Universe, we do not fully understand how they form?
Why do scientists have difficulty understanding how planets form?
In the 20th century, the idea that stars are formed in the middle of giant clouds of gas became an accepted theory. During this process, momentum is conserved and the remnant of a gas cloud collapses into a disc. This is the protoplanetary disk in which the planets are formed.
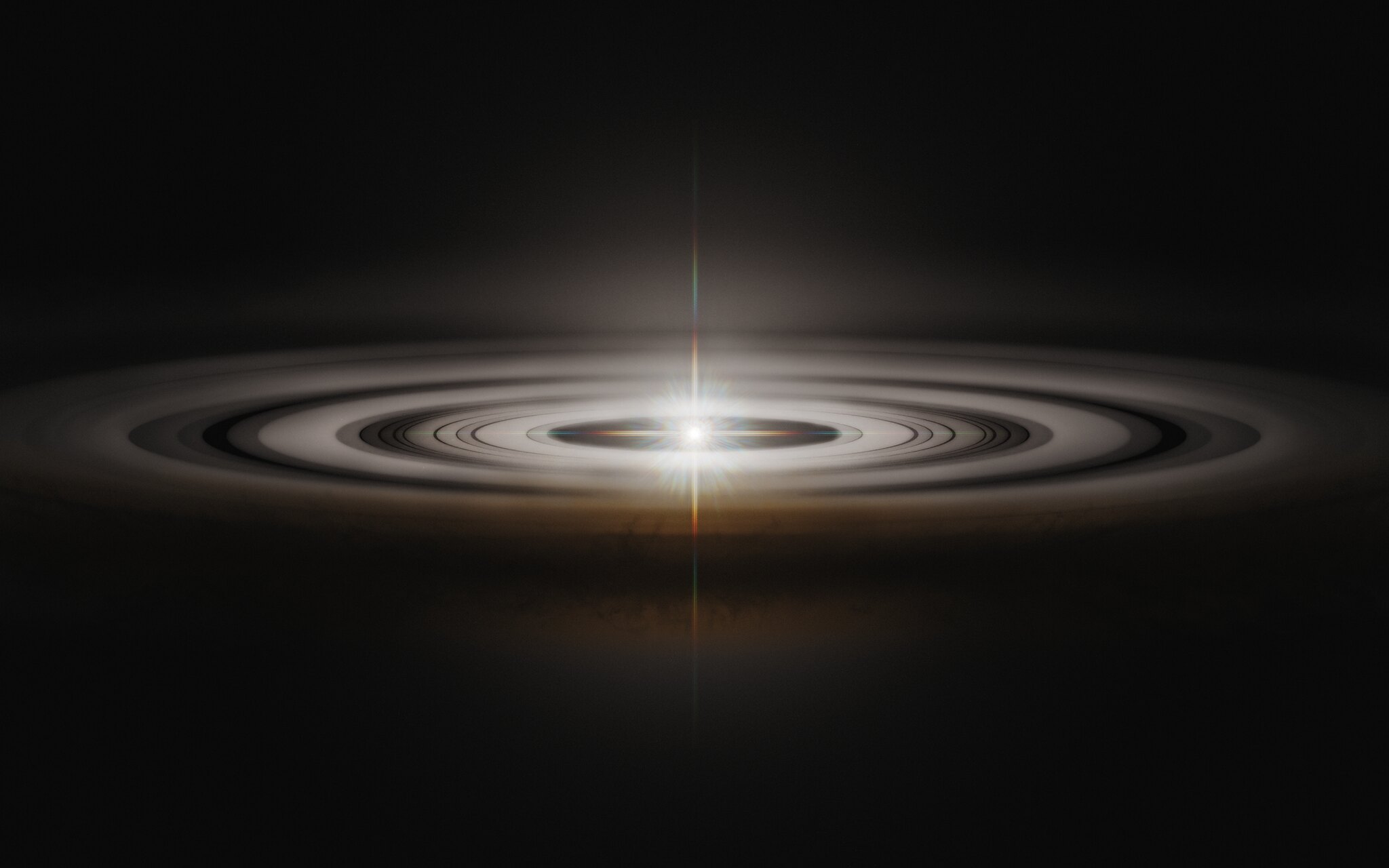
Artistic depiction of a protoplanetary disk (Source: Flickr)
Researchers have come up with different theories on how a planet can form from this remnant material. Our current knowledge suggests there are two different hypotheses to explain planetary formation: one is the theory of gravitational instability, the the other the theory of core accretion. The process of planetary evolution itself is so complex that the behaviour of these contracting gas clouds can only be investigated using numerical simulations, i.e. they can only be modelled using powerful computers. Based on the result of these studies, we now know that the two previous theories are not entirely correct.
A critique of the two previous hypotheses of planetary formation
One important aspect of planetary formation is that protoplanetary discs do not last forever, having a lifespan of generally less than five million years on average. This, therefore, is how long it takes for planets to form. For example, Jupiter's formation required the accumulation of nearly ten Earth masses of solid matter, followed by a gas mantle nearly three hundred times as massive.
In fact, gravitational instability is the same physical process that creates stars. Numerical hydrodynamic simulations have shown that a Jupiter-sized clump of gas cannot coalesce because the gas heats up so much in the process that it does not allow the material to condense further. This means that according to the calculations, the theory is not workable, or only at large distances from the star, due to the physical properties of the protoplanetary disc.
According to the core accretion hypothesis, small particles of dust in a protoplanetary disk are crushed together on impact, and then slowly form planetary germs that later grow into planets. There are several known problems with this hypothesis. One of the biggest is that solid bodies – called planetesimals – that have grown to the size of boulders in the process are rapidly collapsed into the star by the drag of the disk's gas, causing the building blocks of the planets to disappear quickly.
Anticyclonic vortices as planetary embryos
An international team of researchers led by Zsolt Regály has discovered a physical process that could create a multitude of planetary embryos in the protoplanetary disk, providing a possible explanation for the formation of Earth-like planets or giant planets the size of Jupiter. It was already known that protoplanetary disks can form anticyclonic vortices, in which the gas swirls in the opposite direction to the star's orbit. Anticyclonic vortices are very efficient at collecting solid material, which means they produce a higher probability of dust particles meeting and clumping together. Such a vortex also protects the planetesimals that form there from falling into the star, creating ideal conditions for planet formation. But how can such a multitude of vortices form?
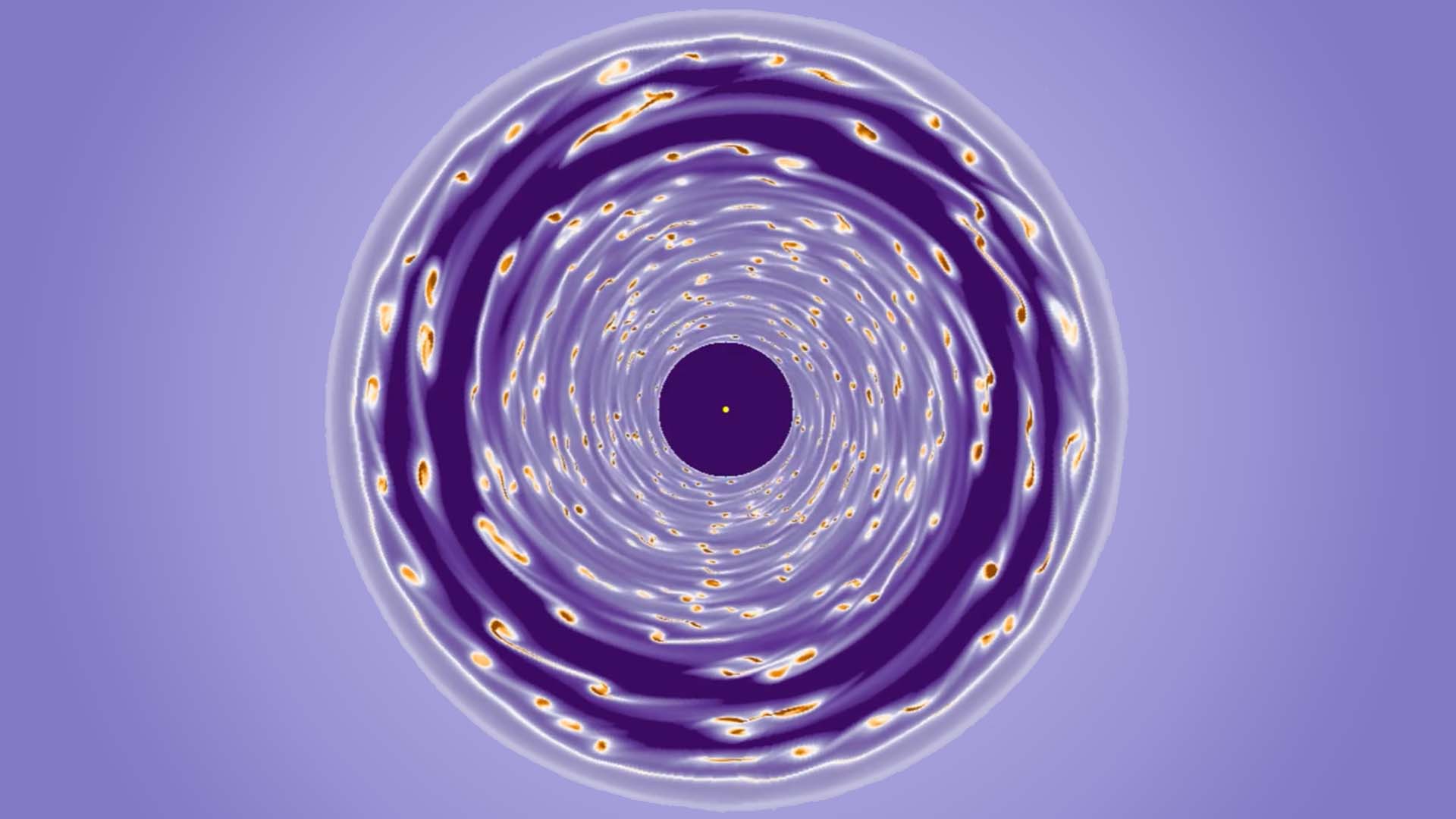
A multitude of vortices in the protoplanetary disk
The dynamics of the gas and dust particles are influenced by the viscosity of the disc, i.e. the degree of internal friction of the matter inside. The new theory posits that the viscosity of the disc depends on the amount of dust in the environment. The reason for this is that the viscosity of the disc is a complex process, formed by the interaction of free ions and the star's magnetic field. The researchers claim that the dynamics of protoplanetary disks are influenced by the dust itself, which can bind free ions in the disk, reducing the viscosity.
If there is a small amount of dust condensation somewhere in the disc, the number of free ions will be reduced and the viscosity will therefore be lower. Where viscosity is reduced, gas dynamics increase the density of the gas, which sucks in the dust, and this reduces viscosity even further. This positive feedback creates small vortices that generate new vortices.
The researchers used two-dimensional numerical hydrodynamic simulations to investigate the number of vortices and and their stability; how much dust they collect. According to the study, the cascade of small vortices creates stable larger vortices that quickly become potential planetary embryos, which could lead to the formation of an entire planetary system. The research has shown that planetary systems can form much faster than previously thought.
The above suggests that this unique discovery may explain the long-studied phenomenon of planetary formation. A more detailed, three-dimensional study of the vortex cascade is still needed before the hypothesis can be fully confirmed. By continuing the project, researchers can also find answers to how the solar system and the distant planetary systems with their very different structures could have formed.